Features
We like to think of a healthy organism as a smoothly running machine. A whole made up of intricately related parts. Right down to the cellular level—and inside each cell itself—every element with its role; every function contributing to the good of the whole.
But if you look deeply enough into living cells, you’re likely to find a whole lot of chaos.
That chaos is something Rochester biologist Jack Werren observed firsthand in the 1980s. While studying entomology at the Walter Reed Institute of Research, he and a colleague discovered a bacteria that altered the sex ratios of insects by killing a large portion of the male offspring, leaving mostly females. Most surprising, they found, was that the bacteria was transmitted from mothers’ cells to their daughters.
“There is a whole class of inherited elements that alter the reproduction of insects in ways that are beneficial only to the element itself, mostly by altering sex ratios,” says Werren, the Nathaniel and Helen Wisch Professor in Biology.
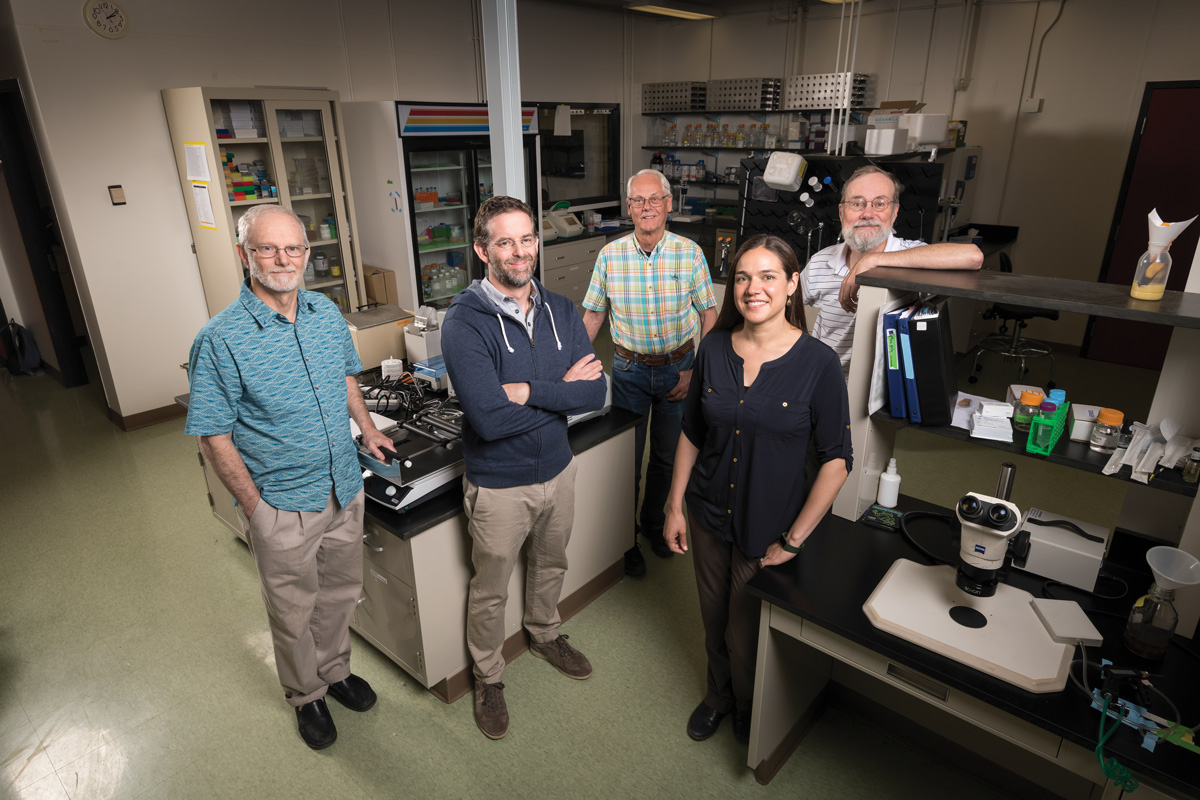
Scientists had observed something similar before. In the 1920s, the Russian biologist Sergey Gershenson first observed a sex-ratio abnormality in a type of fruit fly—an aberration so striking it seemed capable of driving the species to extinction. Barbara McClintock’s seminal work in the 1940s and 1950s had also revealed a world of “jumping genes,” although their “function” in organisms was unclear.
At the time, the implications of McClintock’s research were met with skepticism, but she was later awarded the Nobel Prize. Other mid-century advances led to more puzzling findings. In the early 1980s, right about the time Werren began his Army post, researchers reported that some genes might not have specific purposes, introducing the idea that simple organisms—like onions, which have 15 times more DNA than humans—can have complex genomes, full of repeated DNA. Two influential papers in the journal Nature proposed the idea that repetitive DNA could be parasitic or “junk” DNA.
In 1988, Werren and his Rochester colleagues Uzi Nur and Chung-I Wu brought the diverse discoveries together, formally defining DNA parasites and junk DNA as “selfish genetic elements” (SGEs), an umbrella term for elements that share a common feature: they seek only to enhance their transmission to the next generation and are either harmful or neutral to an organism.
The concept of a selfish genetic element was controversial. It wasn’t just the notion that genes, rather than organisms, competed for survival. That idea wasn’t new. In the 1970s, Richard Dawkins had popularized much of the science of the so-called gene-centric view of evolution in the book The Selfish Gene. But Dawkins’s selfish genes, infighting for their own survival, also helped perpetuate the individuals carrying them. In that schema, every gene in an organism was a selfish gene.
What Werren and his colleagues were describing was something else: genetic elements whose successful propagation was often harmful to an individual and could lead to a species’ end.
“Many biologists had a hard time wrapping their head around the idea that there could be parasites in the DNA,” he says. “If there was something there, it had to be good for the organism.”
Today Werren is one of a group of researchers in the University’s biology department studying SGEs and their impact on evolution.
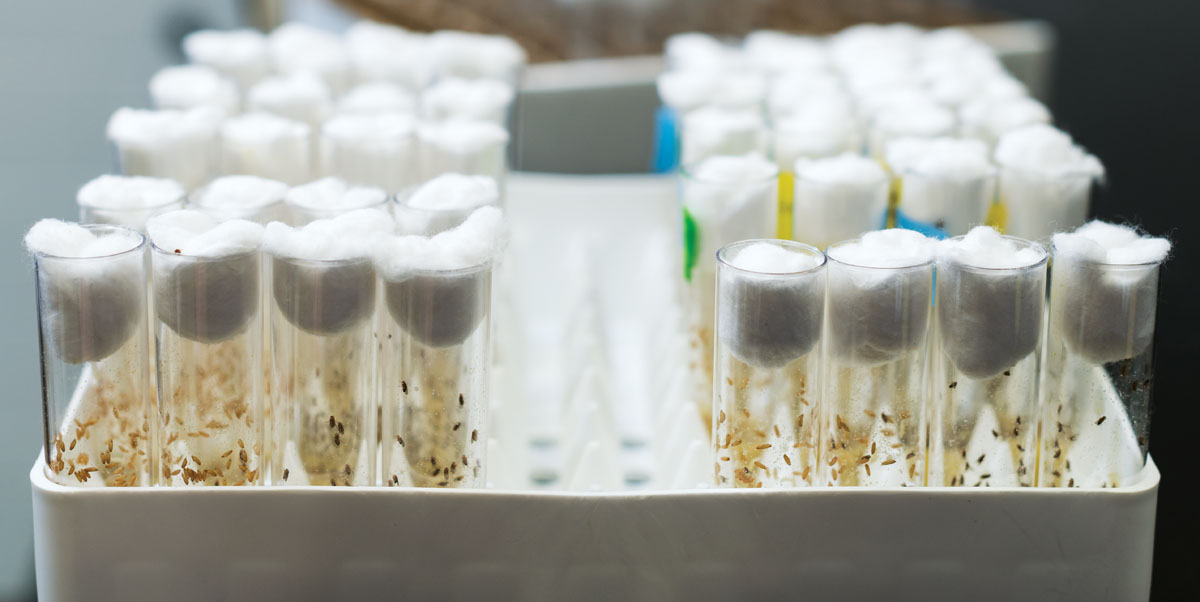
“The University of Rochester has one of the highest concentrations of researchers working on selfish genetic elements in North America, if not the world,” says Arvid Agren, the Wenner-Gren Fellow in the department of organismic and evolutionary biology at Harvard University. “For many years members of the department have shaped the field, and they continue to do so.”
Rochester biologists have been instrumental, for example, in discovering just how significant the elements really are. Organisms from corn to fruit flies to humans are littered with them, affecting a range of biological processes, including speciation, aging, diseases, gene regulation, and even sex itself.
“Something very dramatic has shifted,” says Daven Presgraves, a Dean’s Professor in the Department of Biology at Rochester, who studies the role of SGEs in speciation. SGEs “aren’t just these one offs that you can find here and there. Selfish genetic elements are everywhere. And they’re affecting every generation, all the time.”
A Closer Look at Selfish Genes
Each of the cells in your body shares the same sequences of DNA, collectively called your genome. Each of the genes that make up the DNA, however, is coded to activate different sequences of proteins, giving cells various functions
It turns out, however, that a good portion of your DNA is not even “yours.” That is, it doesn’t code for anything that makes you, you.
Before DNA sequencing, it was difficult for researchers to grasp that a genome is not always a cohesive unit working to optimize an organism’s fitness. When Charles Darwin first articulated the theory of natural selection, he had no notion of genes and DNA or the role they play in inheritance.
In the mid-20th century, researchers began recognizing that DNA held the genes that were passed on from parent to offspring. The era of molecular genetics had arrived and with it, the realization that genes were the vehicles of evolution.
Scientists now know that SGEs can be grouped into several different categories. So-called transposable elements, which make up at least 50 percent of the genes in the human genome, are one major group. Also called jumping genes or mobile elements, they gain a replication advantage by making extra copies of themselves and inserting themselves into random parts of the genome. In so doing, transposable elements are akin to viruses. But unlike viruses, they cannot leave the cell; instead, they infect a cell’s DNA.
“You can basically say that most of the human genome is just old copies of mobile elements,” says Tom Eickbush, a Mercer Brugler Distinguished Teaching Professor at Rochester.
Transposable elements are found in all species of plants and animals. Working with fruit flies, Eickbush has studied the mechanisms by which transposable elements replicate themselves. Their presence “can play a significant role in altering the size, structure, and expression of a cell’s genome,” Eickbush says. Transposable elements can introduce genetic mutations resulting in predispositions to cancer and genetic disorders like hemophilia, for example.
Meiotic drive elements are another major grouping of SGEs. Meiotic drive elements are chromosomes that gain a transmission advantage by getting passed on to more than their “fair” share of offspring. In the regular course of reproduction, parents contribute DNA to their offspring by packaging one copy of each chromosome into gametes (eggs for females; sperm for males) during a cell-division process called meiosis. The production of gametes ensures that each copy of a chromosome makes it into 50 percent of the gametes. Each gene, then, should have the same chance as any other of transmitting itself.
But there are “cheater” genes that skew the ratio. Richard Lewontin, who was an associate professor of biology at Rochester in the late 1950s and early 1960s, developed one of the first models for SGEs, working with the meiotic drive element t-allele, in mice. Lewontin conducted simulations showing that t-alleles—so named because the laboratory mice strain with the cheating allele was tailless—kill gametes carrying the opposite allele and can ultimately drive mice populations to extinction.
Even skewing the ratio a little bit can therefore make a huge difference, says John Jaenike, a professor of biology at Rochester. “Any gene that can cheat during meiosis instead of having a 50/50 segregation—even if it can get 53/47—gains an advantage and that kind of ratio would be difficult to detect. It could be that a lot of genes spread and we don’t even notice it.”
Jaenike studies a destructive inheritable bacteria called Wolbachia, which is widespread in insects, inherently found in 40-70 percent of all insect species. Wolbachia is an example of a third type of SGEs called heritable microbes, which distort sexual reproduction by favoring the sex that transmits them. For instance, males can be infected with Wolbachia, but do not transmit it. Wolbachia therefore distorts reproduction to enhance the chance that a mother will produce female offspring that will then pass on the bacteria.
In his early years at Rochester, Werren observed a fourth type of SGE that takes selfishness to excess: postsegregation distorters. Postsegregation distorters have been detected in worms, insects, yeast, and bacteria and act after fertilization to either kill the genome or the offspring that don’t carry them. Werren observed a type of post- segregation distorter called paternal sex ratio (PSR) in the parasitic wasp species Nasonia vitripennis that completely obliterates a male’s genome, destroying all other genes except its own from the wasp’s sperm after the sperm fertilizes an egg.
“This is the ultimate selfish gene,” Werren says. “You can’t get much more selfish than totally destroying your host genome and getting transmitted yourself.”
How “Selfish” Is the Human Genome?
It’s difficult to directly determine just how much of the human genome is selfish. When researchers map the human genome, they can directly see transposable elements—they are simply pieces of the genomic puzzle that don’t fit. But when it comes to meiotic drive elements, researchers often look for indirect evidence of the cheaters.
Gregor Mendel’s laws of inheritance dictate that a set of parents should produce offspring at a ratio of 50 percent male and 50 percent female. One way to infer the presence of SGEs like meiotic drive elements, which involve the X and Y sex chromosomes, is to observe generations of an organism and see if their sex ratios are highly distorted: does a set of parents have a lot more male offspring than females and vice versa?
This question can be difficult to answer in organisms like humans that do not produce many offspring. “You can think off the top of your head of families that have five or more girls, and that can happen randomly,” says Amanda Larracuente, an assistant professor of biology at Rochester, who studies Y chromosomes and blocks of repetitive DNA called satellite DNA.
On the other hand, “if you have a family pedigree that for generations had individuals that consistently produced only female offspring, one possibility is that there is a driver on the X chromosome that kills Y-bearing sperm,” she adds. This phenomenon is well studied in some fruit fly species.
A successful driver that occurs on the sex chromosomes can be “really harmful for a population,” Larracuente says. A successful driver on the Y chromosome would produce a population of all males, just as a successful driver on the X chromosome would produce all females. “A population like that will collapse and go extinct.”
“We’re Really Only Beginning”
While the proliferation of SGEs is apparent, where they first originated is still a mystery. What is clear, however, is that they do not have a master plan to take over an organism—genes aren’t forward looking.
“Evolution doesn’t know where it’s going,” Jaenike says. In other words, while SGEs are operating only to propagate themselves, they don’t “realize” that by doing so, they may be on a path that could eventually take their host species—and themselves—to extinction.
But genomes do fight back. Within the genomes of every organism there is a microscopic evolutionary arms race taking place: SGEs present themselves in the genome, and the rest of the genome evolves suppressors to quell them. The SGE then evolves to overcome the suppressor, and the suppressor has to evolve to keep pace.
While researchers previously focused on discovering the different types of SGEs and their means of propagation, the field has turned toward understanding this evolutionary arms race.
“Now we are in the genomic sphere,” Eickbush says. With enhanced sequencing technology and new genome assembly methods, “people are looking at total genomes and characterizing the millions of elements that might be there. There’s a great interest in finding out how these elements have given rise to steps in evolution and how they are being coopted for other purposes.”
As a result, biologists in a wide range of subfields have begun contending with SGEs.
Rochester biology professor Vera Gorbunova, for example—whose research is focused on cancer and aging—recently discovered a defense mechanism against SGEs that may trigger inflammation, hastening the aging process in humans.
Larracuente has found that some of the genetic elements may have both selfish and beneficial purposes. Using genome sequencing to map the genome of the fruit fly Drosophila melanogaster, she discovered transposable elements that came and “sat down” next to genes, and the genome repurposed the transposable elements to build essential chromosomal regions.
SGE research is also being applied to synthetic drive systems that could be used to suppress pest populations. Wolbachia, for example, spreads through insect populations by altering reproduction and, as such, is one of the most “successful” SGEs. But it also suppresses some viruses: if a mosquito carries certain strains of Wolbachia, it won’t transmit dengue fever and malaria. Injecting mosquitoes in specific regions with Wolbachia and allowing it to spread through a population can therefore help reduce the spread of disease from mosquitoes to humans.
These methods of using SGE mechanisms to create synthetic drive systems are studied under controlled circumstances—in sealed buildings, for instance—so scientists can test and predict how gene drives might affect ecosystems. Researchers can then develop strategies to minimize risks, including preventing the elimination of an entire species.
“This is reviewed really well in terms of people thinking about how to do this responsibly,” says Larracuente, who regularly discusses her research with synthetic biologists.
By better understanding the different types of SGEs, the ways SGEs spread, and how they give rise to steps in evolution, synthetic biologists are better able to weigh the risks and benefits of creating engineered systems.
But, while SGEs are now almost universally accepted as drivers of evolution, there is still more work to be done.
“We’re really only beginning to dig in to understand selfish genetic elements as a phenomenon,” Presgraves says. “We now assume that at any point in transmission, there’s a selfish genetic element looking for a way to exploit the transmission. That’s how pervasive selfish genetic elements are.”