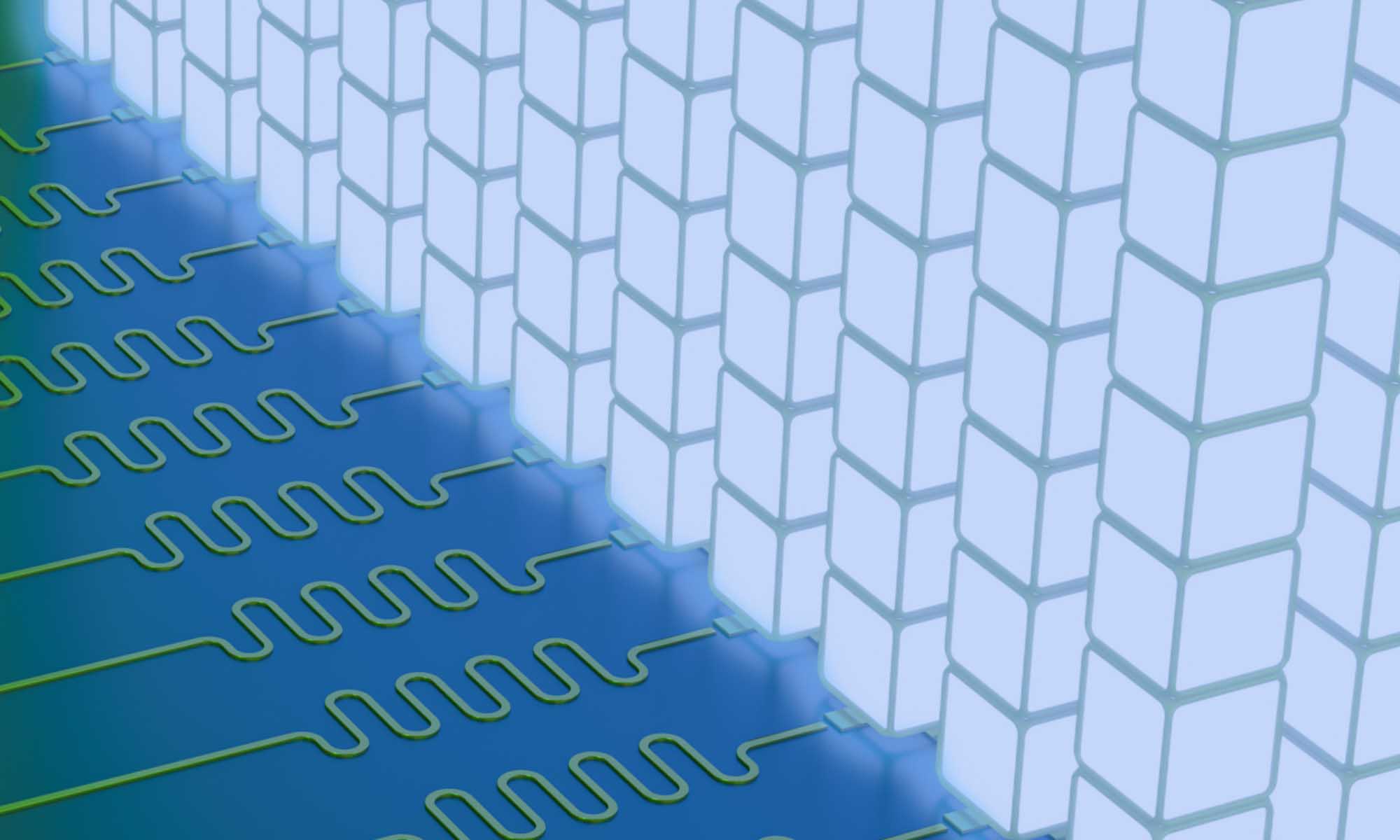
Science & Technology
Researchers discover more efficient way to route information in quantum computers
Using qudits, Rochester scientists have solved a notoriously difficult problem involving Hilbert space, or the quantum matrix.