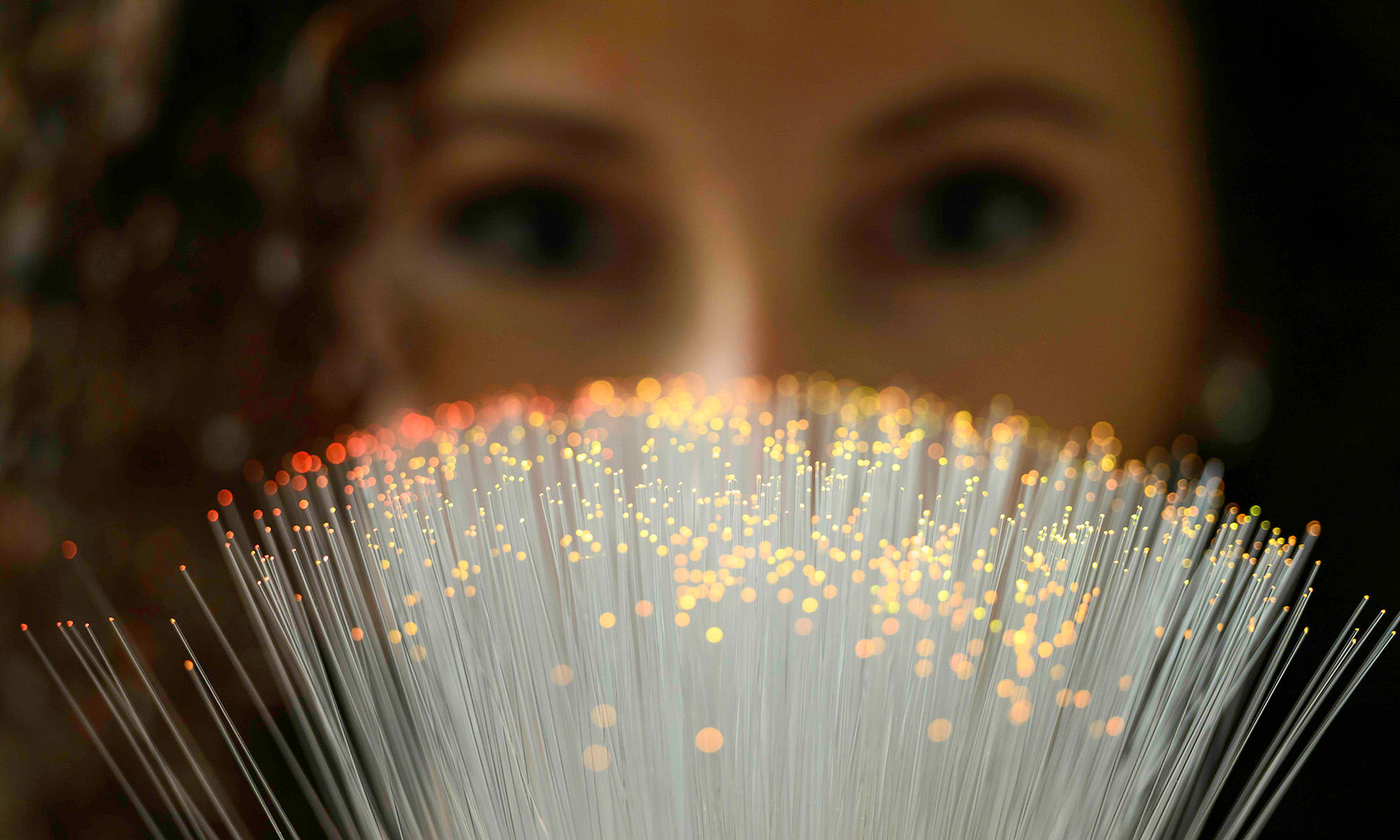
Science & Technology
The surprising way that fiber optics connects us
An optics expert explains how thin strands of glass that transmit light make modern telecommunications possible.
Rochester researchers on the growing concern of plastics exposure on human health.