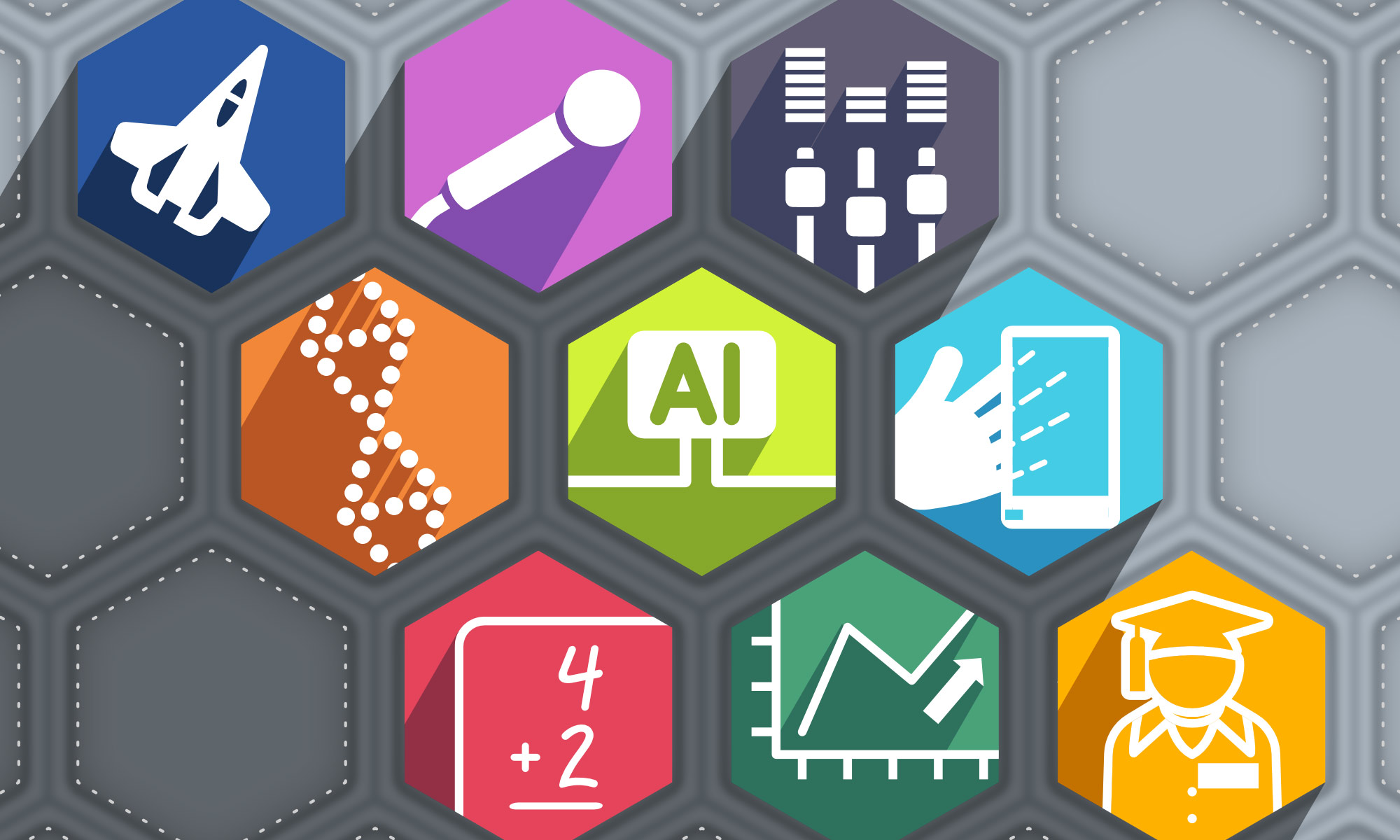
Campus Life
Back-to-school meets future-ready: New academic programs at Rochester
The range of recently launched degree programs reflects what students, the workforce, and the world need and want to become ever better.
A pair of Rochester historians are chronicling the history of the world’s glacial regions—and human responses to their rapid disappearance