The force seems to be with Kevin McFarland.
The question is, "Which force?"
For the assistant professor of physics and astronomy, and an expert on
the forces that bind together particles so small that they are almost
easier to imagine than to calculate, the question vibrates on as many
levels as the most hyperactive valence electron:
Has McFarland and his colleagues on a 40-person research team discovered
a new physical force-one that operates at distances of 1/1000th of an
atom's nucleus and requires energies on the order of 100 million watts
to replicate-during experiments with subatomic particles known as neutrinos?
If so, will the force of history prove the discovery to be a statistical
quirk, or instead, an important pit stop on the road to the holy grail
of physics, the unified theory?
"Our results are like a road sign that says, 'Interesting attraction
ahead' as you drive down the thruway," McFarland says.
"It could be the world's biggest ball of twine, and you have to
pay $5 to see it and be disappointed, or it could be a breathtaking vista
that you will remember forever."
Breathtaking, indeed.
While it may be some time before the final verdict is in, McFarland's
announcement last fall that he and his colleagues at Fermilab, the world's
highest energy particle accelerator, had uncovered a surprising discrepancy
in the standard theoretical model-a well-tested explanation of the way
subatomic particles interact with one another and in the way that the
particles are influenced by the known fundamental forces-has set off a
charged debate among particle physicists.
The discrepancy seems slight to non-statistical minds-roughly a 1 percent
difference between theory and result-but that's like the difference between
planning a trip to Iceland and landing on Greenland when it comes to the
ultraprecise world of high-energy physics.
As a Department of Energy description puts it, the results are "the
kind of inconsistency that makes the hair stand up on the back of a physicist's
neck because it could be the first sign of something that profoundly changes
the picture of nature."
For roughly 30 years, that picture has remained fairly stable. Based
on years of high-energy experiments and detailed tests of theoretical
predictions, physicists have developed a subatomic outline of nature that
organizes fundamental particles and forces into a well-defined table of
elements. Each particle and force is believed to have a predictable relationship
with other particles and forces.
And then McFarland's team fired up the Department of Energy's TeVatron
accelerator at Fermilab outside Chicago to conduct a precise test of the
behaviors of neutrinos, an experiment that would ultimately take eight
years to complete and result in the most precise measurement ever taken
of neutrino interaction.
Distant cousins of electrons, neutrinos fall into a branch of the subatomic
particle family tree known as leptons. Electrically neutral, they are
influenced only by what is known as the "weak nuclear force,"
a force that operates only within an atom's nucleus and is responsible
for the radioactive decay of some nuclei.
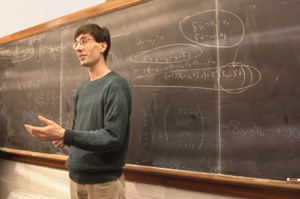 |
Kevin McFarland, assistant professor of physics and astronomy, and
colleagues at Fermilab have completed the most precise measurement
of neutrino interaction ever taken. For more information, visit www.pas.rochester.edu/~ksmcf/NuTeV. |
For comparison purposes, the "strong nuclear force" that binds
quarks-the subatomic particles that make up protons -is so strong that
physicists have never seen one quark separate from other quarks. In contrast,
neutrinos stream through matter as if it were transparent. They are difficult
to pinpoint because they are always free of other particles.
Because of their elusive nature, neutrinos have been something of a mystery
for scientists. Physicists first began speculating about their existence
and their properties in the '30s.
Starting in the late '60s, thanks to work by scientists such as Masatoshi
Koshiba '55 (PhD) at the University of Tokyo, physicists began to piece
together a more accurate picture of the particles.
And, although neutrinos have been part of the universe since the big
bang, the particles continue to puzzle physicists, McFarland says.
"There are two things that we don't know about neutrinos that we
think are fundamental to understanding them," he says. "We don't
really know anything about their size. We know they have a mass, but we
don't know why they have the mass they have. We do know that neutrinos
are, by far, the lightest of the fundamental particles.
"And we don't know anything about their structure," he says.
"But as far as we know, they are fundamental-they can't be broken
apart."
Why the mystery? Part of the difficulty in understanding neutrinos is
that, as with all particle physics research, as the search focuses on
smaller and smaller particles, scientists need ever-higher levels of energy
to separate particles from the forces that hold them together.
And high energy means big equipment, such as the TeVatron accelerator
at Fermilab.
For the neutrino experiment (nicknamed "NuTeV" for "Neutrinos
at the TeVatron"), McFarland's team aimed a beam of protons with
an energy comparable to a gas heated to a billion trillion degrees centigrade
through the NuTeV detector-a 120-foot-long, 700-ton target composed of
alternating layers of detector and steel-and calculated the ratio of high-energy
collisions between neutrinos and other particles.
With the theoretical prediction for that ratio in hand, McFarland's team
compared their results with the predictions from the standard model and
came up with the 1 percent discrepancy.
As McFarland points out, that may not seem like much, but the precision
of the measurement means there is only a 1-in-400 chance that the standard
model is correct in its prediction for neutrinos.
To put it another way, the results indicate that there is a 99.75 percent
chance that neutrinos do not interact as the standard model predicts.
"Our immediate thought was, 'Oh no, we did something wrong,' "
McFarland says.
But no matter how many times the team recalculated-at one point, they
tried to hide the results from themselves to make sure they were being
unbiased -the outcome was the same.
"We were, in fact, testing a textbook definition," McFarland
says. "And then we pushed the definition to a level of accuracy it
hadn't been pushed to before, and-lo, and behold!-we found something different."
For such a weighty breakthrough, the physicist who left a fellowship
at Fermilab in 1998 to join the tenure track at Rochester is self-effacing
about where the discovery might fall in the non-scientific picture known
as "the grand scheme of things."
A comparable measurement of neutrino behavior by experimenters using
an accelerator at CERN in Switzerland and work by others at Fermilab are
offering some support that McFarland's team is on to something previously
unnoticed in particle physics.
As news of the findings rippled through the particle physics community
last winter, physicists were not shy about offering alternative explanations.
A statistical fluke, some said. Others suggested that McFarland had found
confirmation for a long-hypothesized carrier for a new "weaker"
nuclear force.
As yet, nobody is sharpening a pencil to draft a new standard model.
But whether the results indicate a new particle, a new force, or a new
way of understanding the makeup of the atom and the nature of the universe,
McFarland is as excited about the possibilities as when he first added
physics to his double major (with mathematics) as a college sophomore
at Brown.
"My bias is that there is something going on that nobody has thought
of before," he says. "It seems to me very unlikely that the
theory that has worked so well for all these other particles suddenly
fails with neutrinos."
Whether he ends up in the history books as someone who helped piece together
the unified theory doesn't carry a lot of weight for him.
"Who knows, if in the end, people will say, 'It was that thing we
saw at NuTeV?"
"The way basic science works is, it's never just one hint or one
idea," he says. "Fundamental changes in our understanding of
physics often come after we have lots of hints that all point in the right
direction."
"It's always easy to look back 50 years and say, 'That led to this,'
" McFarland says. "It's not so easy to do the opposite, to look
at a discovery and see its path into the future."
Scott Hauser is editor of Rochester Review.
|