Features
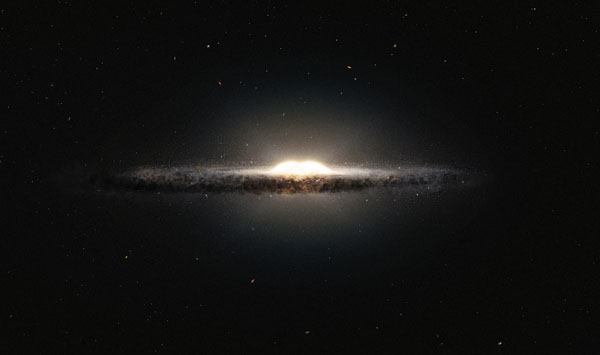
Consider the center of the Milky Way.
More than 24,000 light-years from the sun—far beyond the stars that make up the summer constellations of Sagittarius and Scorpius—the center of our spiral galaxy is home to a dense concentration of stars that date to within a few billion years of the birth of the universe.
Those ancient stars reside in an astrophysical neighborhood known as a “bar” because of its rectangular shape that measures roughly 10,000 light-years in length. And within the bar, orbiting a powerful source of energy believed to be a supermassive black hole, the stars form a bulge that rises vertically to the plane of the bar.
If it were possible for observers to situate themselves on the edge of the galaxy, at eye-level with the horizon of the spiraling cosmic dust, and to look back toward the center of the Milky Way, they would see that bulge, higher at each end and taking a slight dip in the middle, like an astrophysical saddle-back ridge.
Or, as Alice Quillen, professor of physics and astronomy, likes to think of it: shaped like a peanut shell.
How did the bulge get its shape?
That’s a question Quillen, along with an international team from the Sydney Institute for Astronomy in Australia, the Astronomy Institute in Germany, the Shanghai Astronomical Observatory in China, and the Paris-Meudon Observatory in France, addressed in a paper published late last year in Monthly Notices of the Royal Astronomical Society.
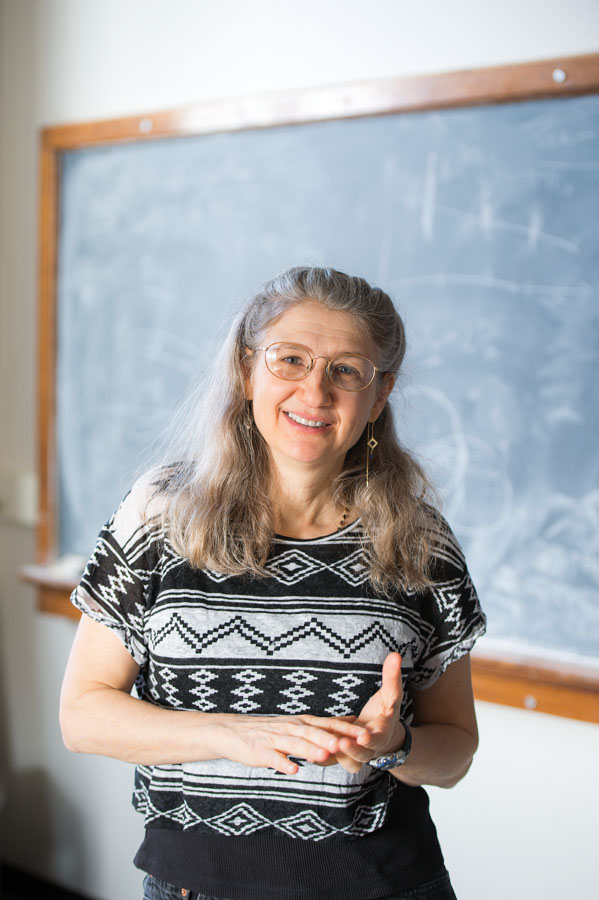
The team reported results of a new mathematical model that suggests the stars move in peanut shell– or figure eight–shaped orbits. Where previous explanations envisioned a relatively straightforward orbital journey—often described as banana-shaped—the new model indicates a more complicated pattern.
The difference is important: astronomers develop theories of star motions not only to analyze how the stars in the galaxy are moving but also to understand how our galaxy—and others like it—formed and evolved. The paper came out as the European Space Agency was preparing for the December launch of the Gaia spacecraft, which is designed to create a 3-D map of the Milky Way’s stars and their motions. The map will help astronomers better understand the composition, formation, and evolution of the galaxy.
“It is hard to look back into the past of our galaxy and know what was there, but simulations can give us clues,” says Quillen.
Unlike the solar system, where most of the gravitational pull comes from the sun and is simple to model, describing the gravitational field near the center of the galaxy—where millions of stars, vast clouds of dust, and even dark matter swirl about—is a more daunting challenge.
In their work, Quillen and her colleagues focused on the forces acting on the stars in or near the bulge. As the stars go through their orbits, they also move above and below the plane of the bar.
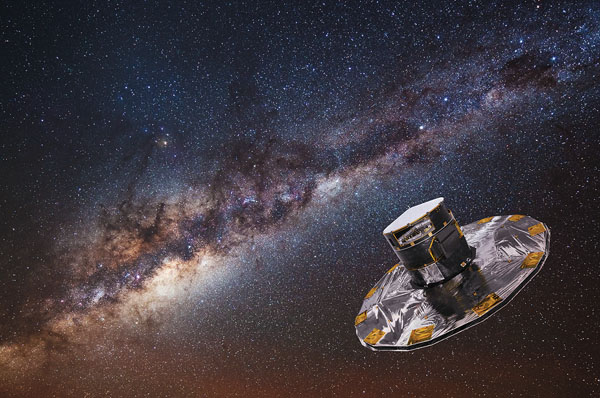
And like a child on a swing, each time a star crosses the plane of the bar at what’s known as the resonance point, the star gets a little push that moves it a bit higher above the plane, forming the edge of the bulge. Through computer simulations, the researchers demonstrated that between the two vertical oscillations of each orbital period, the stars follow orbits consistent with the effect of the resonance, giving rise to the observed shape of the bulge.
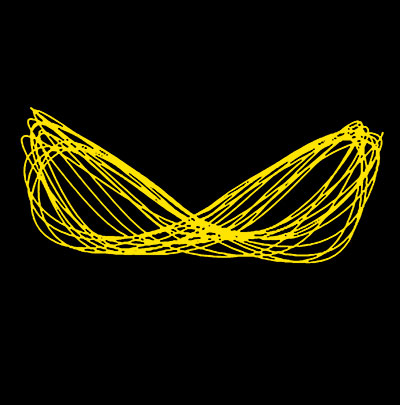
“This may be what happens in our galaxy,” says Quillen.
Quillen says astronomers are interested in finding out how much the bar has slowed over time and whether the bulge “puffed up all at once or slowly.”
Understanding the distributions of speed, direction, and velocity of the stars in the bar and the bulge might help determine the galaxy’s evolution.
“One of the predictions of my model is that there is a sharp difference in the velocity distributions inside and outside the resonance,” Quillen says. “Inside—closer to the galactic center—the disk should be puffed up and the stars there would have higher vertical velocities.”
One of the goals of the Gaia spacecraft will be to measure the motions of the stars and allow astronomers to look for such variations in velocity.
“Gaia will generate huge amounts of data—on billions of stars,” says Quillen, noting that the data will allow her and her colleagues to further fine-tune their model.
“This can lead to a better understanding of how the Milky Way might have evolved into the shape it has today.”
Leonor Sierra writes about the sciences for University Communications.