Overview
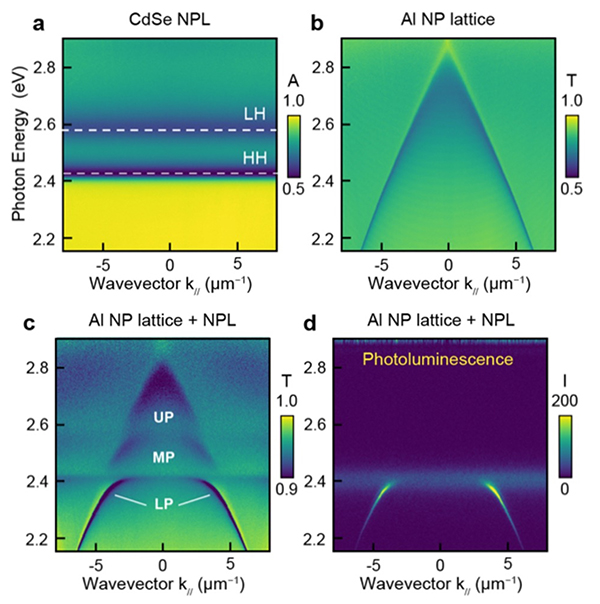
Quest. (n) a journey or effort in pursuit of a goal (often lengthy, ambitious, or fervent); a mission.
QuEST. (n) an NSF-funded Phase I Center for Chemical Innovation to discover a new tool that will enable new chemical reactions that are not currently possible; a mission.
QuEST will explore chemical reactions with polaritons, which arise from molecules strongly coupled to an optical cavity.
Objective 1: Better Cavities for Polariton Chemistry
Challenge: to design accessible optical cavities that can facilitate exciton-polariton formation at room temperature
Cavity design considerations
- Large cavity quality factor (Q)
- Small mode volume
- Potential for integration with fluids
QuEST demonstrated strong coupling at 300 K between the heavy and light hole (HH and LH) excitons of CdSe Nanoplatelets (NPLs)(Fig. 1a) and the surface lattice resonances (SLRs) of an aluminum NP lattice (Fig. 1b). The signature of strong coupling is an anti-crossing (or bending) of the energy bands of the cavity photon states when near resonance with the exciton state of the NPL (Fig. 1c). Strong photoluminescence (PL) was also observed coming from the HH lower polariton (Fig. 1d). In an unexpected development, we tailored the position of the lower polariton band and observed ultra-low-threshold polariton lasing from an exciton-polariton condensate (Fig. 2g,h). Our observation of polariton condensation represents a major discovery for a colloidal nanoparticle system in an open architecture nanoparticle lattice cavity.
QuEST is currently exploring the implications of polariton condensates for driving novel chemical reactivity.
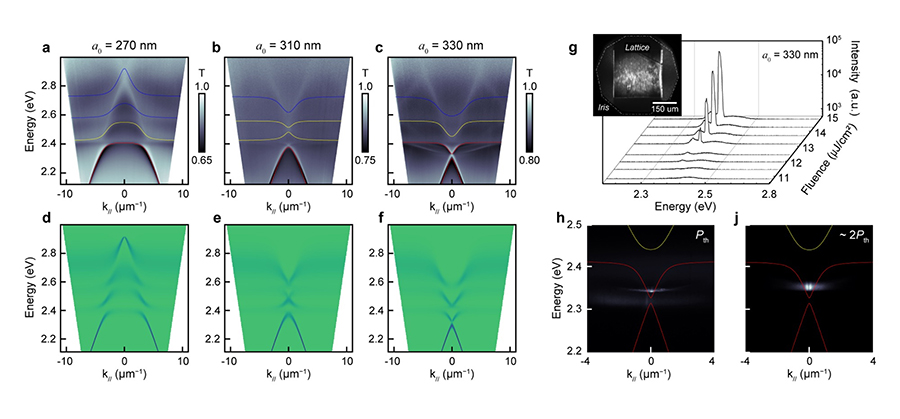
Objective 2: Electrochemical Polariton Characterization
Challenge: determine the absolute energetics of the upper and lower polariton
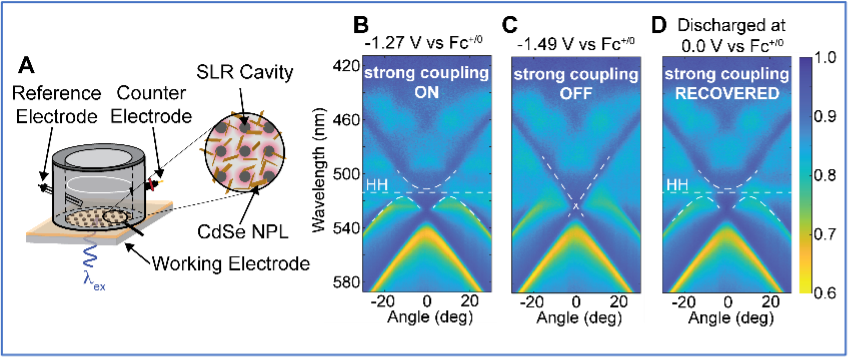
QuEST successfully designed and fabricated a prototype spectroelectrochemical cell that employs the optical cavity working electrode and allows optical access with high numerical aperture microscope objectives (Fig. 3). In a major Phase I breakthrough, QuEST was able to successfully manipulate the strength of the coupling between CdSe NPL excitons and the coupled modes of a metal nanoparticle lattice (Fig. 3) by sweeping the applied potential at the working electrode. Such behavior has not been previously seen in a chemically accessible open cavity system. This Phase I accomplishment firmly establishes that exciton-polaritons can reliably and robustly be placed under electrochemical control, and thus provides a clear path to measure absolute polariton energetics, as well as the exciting prospect of ultimately controlling outcomes of chemical reactions through facile tuning of electrochemical potentials.
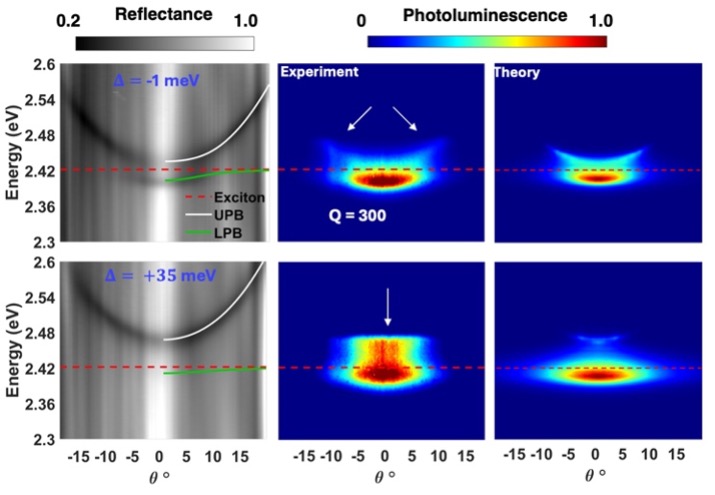
Objective 3: Modify Charge Transfer Rates
Challenge: understand how to use an optical cavity to modify the rate of charge transfer between two molecules
Understanding how to control the temporal decay dynamics for polaritons formed between NPLs and dielectric Fabry-Pérot cavities is important because long polariton lifetimes (relative to the cavity radiative lifetime) will establish timescales for chemical reactivity including charge transfers. In an exciting development, we found that increasing the Quality (Q)-factor 6-fold of our Fabry-Pérot optical cavities resulted in photoluminescence emission from the lower (LP) and upper (UP) polariton branches (LP). (Fig. 4) The observation of PL from the UP is a significant milestone as it is exceptionally rare and indeed represents the first time that such upper polariton PL has been observed in a nanoparticle system. Importantly, state-of-the-art theoretical modeling confirmed that the increase in Q-factor was a primary driver of the observation of PL from the UP (Fig. 4).
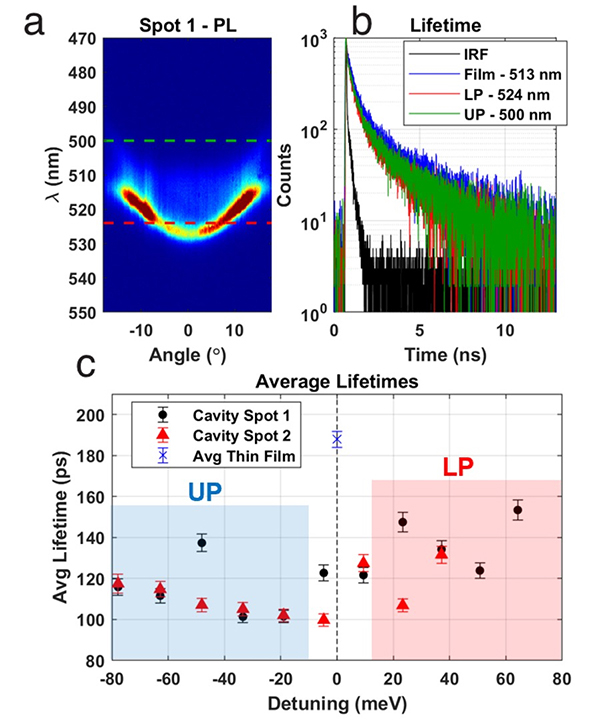
The increase in cavity Q-factor also enabled a clear measurement of the dynamics from the polariton state. As shown in Fig. 5, we found the PL lifetime for the NPL polariton was around 100 ps (depending on detuning, Fig. 5), in contrast to the lifetime of the bare NPL film outside the cavity, which was 190 ps. The measurement of an unambiguous PL lifetime of the polariton state is a crucial achievement for QuEST in the polariton chemistry field. Importantly, we now have a clear timescale for polariton chemistry events (~ 100 ps), which provides ample time for charge transfers to take place, and allows for facile determination of the effect of strong light-matter coupling on charge transfer rates, which is the subject of ongoing QUEST research in this objective.
Objective 4: Modify Chemical Reactions
Challenge: understand how polaritons alter chemical reactivity and selectivity
An overarching goal of QuEST is to develop strategies to use polaritons to change the selectivity of chemical reactions. While formation of polaritons will alter the electron (density) distribution due to the hybridization of light and matter, it is not known in a general way how polaritons modify molecular orbitals, and how those modified molecular orbitals can lead to new chemical reactivity. Without this understanding, establishing general principles for polariton chemistry will be extremely challenging.
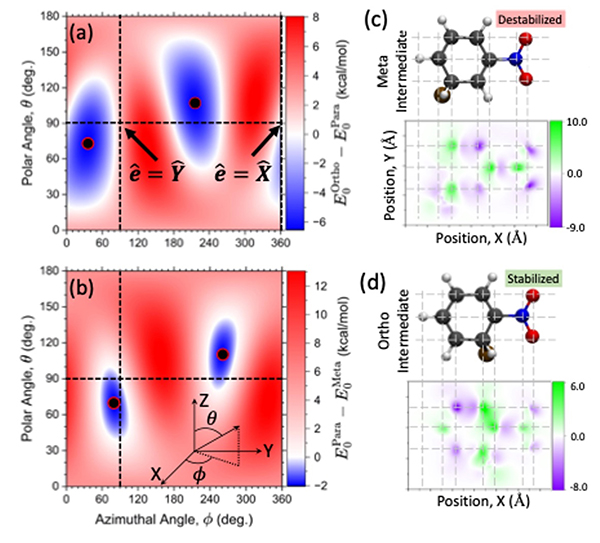
Here, we developed an ab-initio theoretical tool to characterize the change of electron density and transition density when coupling molecules to the cavity [24]. Using this new tool, we have explored how cavities can profoundly influence the bromination of nitrobenzene, which in free space yields only a single product: bromination at the meta position (Fig. 6). However, when a nitrobenzene molecule is strongly coupled to the cavity field, the bromination can be tuned by the polarization of cavity electric field to selectively yield either ortho- or para-functionalization. This exciting result stands in direct contradiction with standard chemical intuition and provides a framework for experimental exploration of exciton polariton chemistry.
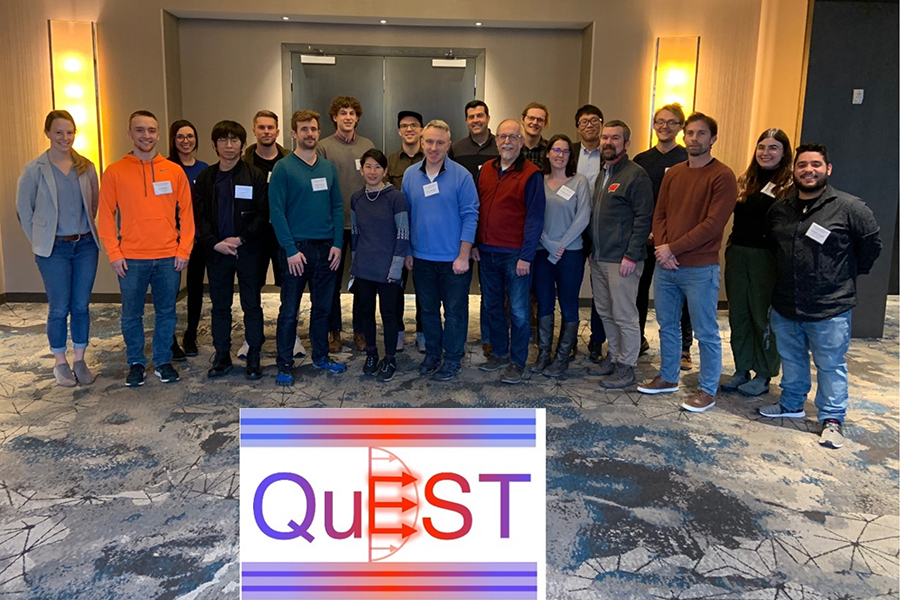